The Cortical Control of Voluntary Movement |
The corticospinal tract controls the movements of the opposite side of the body because its axons cross the midline in the pyramids of the medulla before terminating in the ventral horn. The largest number of corticospinal axons are destined for motoneurones innervating the hands, as shown in the diagram opposite. A large number of cortico-bulbar fibres descend to the motor nuclei of the braintem and are concerned with the muscles of the face, mouth and larynx.
The image shows the areas of motor cortex concerned with motor functions in different areas of the body, and much more cortex is used to control muscles that are involved in fine movements.
While the executive pathway for control of muscles begins in the motor cortex, many cortico-spinal neurones originate in areas in front and behind the pre-central gyrus.
This pathway can be examined in humans using Transcranial Magnetic Stimulation (TMS), described in the section of Clinical Neurophysiology. |
*
|
Functions of the Primary Motor Cortex (MI)
Electrical stimulation of the motor cortex in humans shows that there is a somatotopic map of the musculature on the cortex: stimulation with small electrical currents produces contraction of muscle groups and movements of joints on the opposite side of the body. The pre-central gyrus, the site of the primary motor cortex MI; Brodmann's area 4), is involved in executing movements, and is especially important in the control of fine movements, e.g. of the fingers. The prehensile thumb of primates and humans has a particularly large repertoire of movements, and the largest area of motor cortex is reserved for the fine control of these movements. Each cortical column seems to control of groups of muscles, representing specific movements, rather than individual muscles.
The primary motor cortex (Brodmann's area 4) receives its commands from other areas of the brain concerned with the initiation and programming of movements. Particularly important is the area of cortex in front of the pre-central gyrus, an area known as the pre-motor cortex, and recognised for its role in bringing the motor cortex into action.
|
Top
The Pre-Motor and Supplementary Motor Cortices (Brodmann's area 6)
Brodmann's area 6 is a strip of cortex in front of the primary motor cortex, and comprises the pre-motor cortex, and on the medial side of the hemisphere, the supplementary motor area, or SMA. These areas are important role in the planning of complex, coordinated movements, and lesions of them give rise to a difficulty with initiating movements called Abulia.
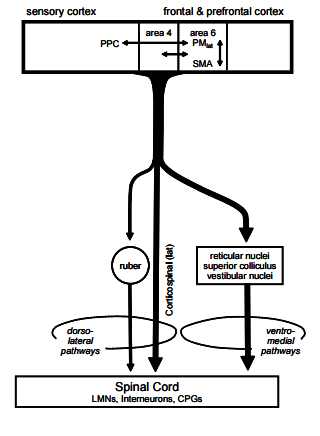
This diagram shows the relationship between the motor cortex and it s adjacent areas, and the descending pathways including the corticospinal tract, and pathways that involve synapses in the brainstem, such as the rubrospinal and reticulo-spinal tracts.
The premotor cortex and the supplementary motor cortex (MII, on the medial side of the hemisphere) have similar functions, and together comprise Brodmann's area 6. Both have connections with the basal ganglia, and all of these become active BEFORE the motor cortex (MI) during voluntary movements, which suggests that they are involved in the initiation and programming of voluntary movements. While MI executes movements, the supplementary and pre-motor areas organise the movement, and bring specific groups of muscles sequentially and smoothly into activity, so as to achieve the objective.
Electrical stimulation of Brodmann's area 6 tends to produce more complex movements than the simple movements that occur when MI is stimulated.
Lesions also give rise to an increase in muscle tone, especially in extensor muscles that cannot be ascribed simply to lesions of the cortico-spinal tract. Alternative pathways involve cortical axons that synapse in the brainstem on bulbo-spinal pathways that are concerned with the regulation of muscle tone.
Bulbo-Spinal Pathways and Muscle Tone |
Bulbospinal Pathways - Overview Regulation of Muscle Tone Reticulo- and Rubro-Spinal Tracts Vestibulospinal Tracts
Functions of these pathways in the regulation of movement and tone |
|
The diagram opposite shows the functional relationships between different prts of the motor control system.
'Other areas of the cortex' include the association cortex that makes contact with the pre-motor and supplementary motor areas, and converge on the motor cortex in the pre-central gyrus.
The thalamus is involved in relaying signals back to the motor cortex from the cerebellum and basal ganglia. The basal ganglia receives inputs from many areas of the cortex; the cerebellum receives inputs from pontine nuclei which relay information form the cortex, and in addition obtain proprioceptive information via the spino-cerebellar tracts.
The motor pathways are (a) direct through the corticospinal tract, and (b) polysynaptic, using corticobulbar and bubo-spinal pathways.
The former executes voluntary movements whereas the polysynaptic pathways are able to execute coarser movements and make adjustments to muscle tone. Tone is dependent on a balance of activities withig different parts of the brainstem, and is also affected through the vestibular system in the control of balance.
Changes in tone involve descending pathways that influence alpha and gamma motoneurones. |
*
|
|
The Frontal Eye Fields are a cortical area within each frontal lobe, which whe stimulated electrically result in the coordinated movements of both eyes in a horizontal direction. These areas correspond with Brodmann's area 8, and receive input from the visual asssociation cortex. They also have connections with the superior colliculi and the paramedian pontine reticular formation (PPRF), a region of the midbrain that has vestibular inputs and is involved in coordinating eye movements during movements of the head. The superior colliculi are involved in initiating changes in the direction of gaze when objects suddenly move into the periphery visual fields, so that the eyes can focus on this object.
|
Balance and Eye Movements
Balance involves inputs from the vestibular apparatus that are used in several ways. One is to influence muscular movements and tone within the body to help maintain the upright posture; and also to position the eyes so that they can fixate on, and follow, an object within the visual field while the head is moving.
The first involves the vestibulo-spinal pathways referred to above; the second involves a brainstem pathway, the medial longitudinal bundle, one on each side of the midline on the floor of the fourth ventricle, which connect the vestibular nuclei with the motor nuclei that control the extraocular muscles (the III, IV and VIth cranial nerves). One area of the pons, the paramedian pontine reticular formation (PPRF) coordinates these movements and has connections with the superior colliculi and the frontal eye fields. More on Balance an Eye Movements
|
|